Looking through the patient’s chart, an infection-control
consultant at the National Institutes of Health noticed something
troubling.
The patient, a 43-year-old woman from New York, was scheduled
to be admitted to NIH’s Clinical Center, a research hospital for people
enrolled in clinical studies and drug trials, in June 2011.
The patient had a rare lung disease, but the consultant spotted
another problem, one that was potentially even more serious: She had been
colonized by a dangerous, drug-resistant bacterium the likes of which the
Clinical Center had never seen before. It was called KPC
klebsiella.
Klebsiella itself is an ordinary bug, one of many bacteria that
reside in our intestines. But bacteria can exchange genetic code with each
other, and this particular strain of klebsiella had acquired a molecule of
DNA known as KPC.
The KPC molecule deactivates carbapenem, an antibiotic that’s
the drug of last resort for bacterial infections. For severely ill
patients, such as many of those at the Clinical Center, the most likely
result of a KPC-klebsiella bloodstream infection is death.
The job of keeping the bug from spreading to other patients
fell mainly to Tara Palmore, the Clinical Center’s deputy hospital
epidemiologist. Although Palmore and other administrators were nervous
about the patient’s arrival, they believed they could contain the
bacterium and prevent an outbreak.
Palmore followed a strict protocol, outlined by the Centers for
Disease Control and Prevention (CDC): Everyone entering the patient’s room
was required to wear protective gloves and gowns. Caregivers were told to
use disposable products whenever possible. These measures, known as
“enhanced contact isolation” would be maintained both in the ICU and later
in the patient’s room.
The patient arrived on June 13, 2011. She spent 24 hours in the
ICU, then was transferred to a private room. Later that month, she
returned briefly to the ICU, again under enhanced isolation. Afterward,
groin and throat cultures were collected from other ICU patients; none
tested positive. On July 15, five weeks after the patient checked into the
Clinical Center, she was discharged. The testing continued for another
week. All results were negative. It seemed the hospital was in the
clear.
Then, on August 5, Palmore got a call from Adrian Zelazny, head
of the microbiology lab. A 34-year-old male cancer patient in the ICU had
developed an infection. It was KPC klebsiella.
Palmore was puzzled. The cancer patient had never overlapped in
the ICU with the first colonized patient. Was this a new, independent
infection, or had the bacterium found a way to hide, undetected, in the
hospital for weeks before spreading to other patients? The microbiology
lab could say only that the two strains were similar.
Ten days later, a third patient, a 27-year-old woman, tested
positive. Within months, both the second and third patients would die from
sepsis brought on by the infection. (NIH declined to provide patient names
for this story, citing privacy concerns.)
And that was just the beginning. Before the outbreak was
over—if it was over—seven other patients would die. “The system broke,”
says Dr. John Gallin, director of the Clinical Center. “Something went
wrong.”
But this isn’t a story about wrongdoing. It would be reassuring
if it were.
For four months, the staff at NIH mounted an extraordinary
effort to contain the outbreak, one that involved both old-fashioned,
epidemiological detective work and cutting-edge genomic science. The story
of that response—and how doctors and scientists at one of the world’s most
advanced medical facilities were bested by a microscopic
organism—illustrates the dangers we face as we edge closer to a future in
which antibiotics no longer reliably work.
• • •
At the Clinical Center’s groundbreaking in 1951, President
Harry Truman declared victory over the terrible infectious diseases of his
childhood—typhoid, diphtheria, tuberculosis, and pneumonia. “It is safe to
say that we have successfully conquered the infectious diseases,” he
said.
He was wrong. Infections in medical facilities now kill some
99,000 Americans a year—more than three times the number of lives lost
every year to car accidents or gun violence. That’s roughly the equivalent
of a fully loaded 737 jet crashing every day and killing every passenger.
Check into a hospital and there’s a 5-percent chance you’ll acquire an
infection. If you do, there’s a 6.5-percent chance you’ll die as a
result.
By overprescribing antibiotics for ourselves and the animals we
eat, we humans have done something foolish—we’ve waged a war on microbes
that has only made them stronger. According to the CDC, more than 70
percent of the bacteria involved in hospital-acquired infections now have
at least some resistance to antibiotics. And the problem is getting
worse.
In March, Dr. Thomas Frieden, head of the CDC, warned of the
dangers posed by KPC klebsiella and other drug-resistant bacteria. “It’s
not often that our scientists come to me to say that we have a very
serious problem and we need to sound an alarm,” Frieden said on a
conference call, “but that’s exactly what we’re doing today.”
Frieden described “nightmare bacteria” that posed a triple
threat: “First, they’re resistant to all or nearly all antibiotics, even
some of our last-resort drugs. Second, they have high mortality rates.
They kill up to half of people who get serious infections with them. And
third, they can spread their resistance to other bacteria.”
That last point is perhaps the most alarming. Think of KPC as a
code that gives bacteria resistance to the last class of antibiotics we
have that can stop infections—a code that klebsiella can share with other,
more common bacteria. That means, Frieden said, “that we have only a
limited window of opportunity to stop this infection from spreading to the
community and spreading to more organisms.”
Beyond that window, things start to look pretty scary. “We have
eight years until 15 percent of the people who come to us have serious
infections that we will not have treatments for,” says Dr. Allison McGeer,
an infectious-disease epidemiologist at Mount Sinai Hospital in Toronto,
which confronted the first SARS outbreak in North America ten years
ago.
“Right now, if you get a urinary-tract infection, the doctor
pats you on the head and gives you an antibiotic,” says Dr. Brad
Spellberg, an infectious-disease expert at UCLA. “People don’t die of
urinary-tract infections. They are going to start to.”
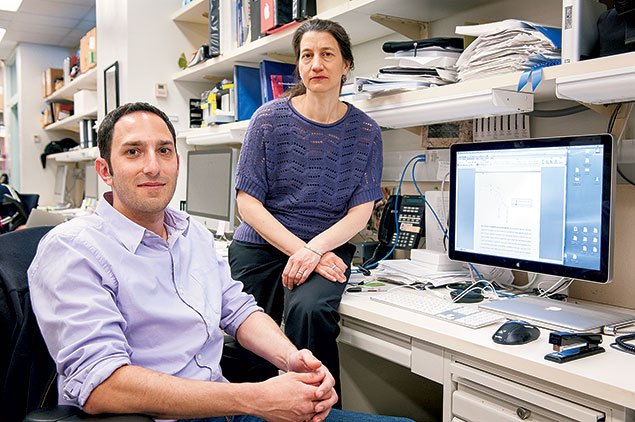
Tara Palmore was 16 years old the first time she watched
someone die. He was a drug user who’d been brought into the emergency room
of DC’s Specialty Hospital on Capitol Hill with multiple stab wounds. She
was a candy striper from Arlington and a student at the Madeira School in
McLean.
From Madeira, Palmore went to Harvard, where she wrote a thesis
on the response of Benjamin Rush—the only Founding Father who was a
physician—to a deadly outbreak of yellow fever in Philadelphia in 1793.
After graduating from Harvard in 1992, Palmore entered medical school at
the University of Virginia.
When asked about the attributes of an outstanding hospital
epidemiologist, Palmore lists three: “biostatistical skills,” “being
detail-oriented,” and, most important, “an open-mindedness about how
things in a hospital work and being able and willing to think beyond the
dogma of the field.”
As Palmore tried to apprehend this strain of KPC klebsiella,
she would need all of these things and one more: a genetic fingerprint of
a sort no hospital epidemiologist had ever had access to before. And for
that, she would need the help of two scientists working across campus in
NIH’s Building 49.
Julie Segre’s job title is senior investigator and head of the
epithelial-biology section in the genetics-and-molecular-biology branch of
the National Human Genome Research Institute. But the most important thing
to know about Segre is this: She is a code breaker.
It runs in the family. Her grandfather was a cryptanalyst for
the Allies in World War II. His brother, Emilio Segrè, won the Nobel Prize
in 1959 for discovering the antiparticle of a proton. Julie Segre’s father
was a theoretical high-energy physicist at the University of Pennsylvania.
(He studied neutrinos.) An uncle and several cousins are also physicists.
“That’s sort of the family business,” says Segre. “Physics.”
After graduating from Amherst in 1987, Segre headed to MIT to
pursue a PhD in genetics. There, as a member of the team working on the
Human Genome Project, she focused on one of life’s greatest puzzles—how
DNA encodes the instructions for life.
In 2009, Evan Snitkin, a 28-year-old postdoctoral fellow from
Boston University, joined Segre’s lab. They began to work on ways DNA
sequencing could be used to combat hospital-acquired
infections.
Think of a hospital dealing with an outbreak as a crime scene.
Epidemiologists traditionally search for the culprit by looking for
commonalities among infected patients. Sometimes they find a smoking gun—a
contaminated respirator, for example. But they’re often forced to make do
with circumstantial evidence. The fact that Clinical Center patients were
testing positive for KPC klebsiella two months after the first infected
patient was admitted raised suspicions that there might have been a
transmission, but the epidemiologists had no proof.
DNA sequencing could remedy that. By culturing bacteria from
each patient and then sequencing their DNA, Snitkin and Segre could
determine whether the Clinical Center was dealing with a single outbreak
or with multiple outbreaks. When the Clinical Center had encountered
Acinetobacter baumannii in 2007, they had done precisely that.
Their analysis had shown that the hospital was dealing with several
strains of the bacterium—not one ongoing transmission. But their analysis
had been conducted retrospectively, well after the hospital had acted to
prevent transmissions.
As part of his postdoc training, Snitkin worked with Adrian
Zelazny in the Clinical Center’s microbiology lab. Zelazny had trained in
Israel, and he told Snitkin about a KPC klebsiella outbreak there that
infected hundreds of patients at several hospitals.
Snitkin realized that he and Segre now had an opportunity to do
something that had never been tried—to use DNA sequencing to understand an
outbreak in real time. Segre told Palmore and her boss, Dr. David
Henderson, that Snitkin was going to analyze the cultures taken from all
of the colonized patients.
On August 23, 2011, a 29-year-old man with lymphoma tested
positive for KPC klebsiella. Six days later, another colonized patient—a
54-year-old man with a tumor—was identified. The Clinical Center now had
five infected patients.
Henderson and Palmore had no idea where the infections were
coming from or how they were spreading. All they knew was that they needed
to stop them. Patients who tested positive for KPC klebsiella were moved
out of the main ICU into a six-bed unit across the hall. A separate
isolation ward was set up for those patients after their discharge from
the ICU. Nurses, physical therapists, housekeepers, social workers—anyone
who worked with colonized patients—would deal only with those patients
until the outbreak was under control. The Clinical Center hired 15 outside
“adherence monitors” to ensure that hands were washed, visitors were
gloved and gowned, and hand sanitizers were used properly (two pumps, not
one).
Some of the patients had recently undergone chemotherapy, which
devastated their immune systems. Others had breaks in the lining of their
intestines that gave the bacteria easy access to their bloodstream or had
spent considerable time on ventilators and central-line catheters, which
provide another pathway for bacteria to enter the blood.
The pattern was heartbreaking. Patients with infections were
given a cocktail of three antibiotics—colistin, tigecycline, and
gentamicin. These treatments were themselves toxic. Colistin had been
largely abandoned in the 1960s because of the damage it causes to the
kidneys. Often the patient would rally, but only for a few days. In fact,
what the cocktail of drugs was doing was killing off the least resistant
bacteria. Then the infection would return in a more virulent form. At that
point, Palmore says, “there was almost nothing left we could do, nothing
we could treat.”
• • •
Snitkin was racing to analyze the bacterial DNA taken from the
first five patients. On September 14, he got the sequences for each of the
five samples from the NIH Intramural Sequencing Center in Rockville. It
was now a matter of running computer programs he’d written earlier to sort
and compare the results. He told Segre and Palmore he’d have his initial
analysis by 2 pm the next day.
Segre and Palmore arrived at the conference room on Building
49’s third floor the following afternoon. A few minutes later, Snitkin
walked in, looking tired but excited.
“Here’s what we see,” he began. “There are fewer than ten
differences across the entire 5 million base pairs of each genome
separating patient one’s genome from patients two, three, four, and five.”
In other words, the DNA taken from the first five patients was virtually
identical.
“Patients two through five are definitely related to patient
one,” Snitkin said. That meant Palmore and Henderson were dealing with a
single strain.
It was also possible to deduce the order of transmission from
the mutations that had taken place. Henderson and Palmore had puzzled over
how the KPC klebsiella had moved from patient one to patient two, given
that the two patients had never overlapped in the ICU. Snitkin and Segre’s
analysis suggested a simple answer: It hadn’t. Instead, patient one had
transmitted the bacterium to patient three who, while infected but not
symptomatic, had passed it to patient number two.
For Palmore, it was a eureka moment. The Clinical Center was
dealing with one continuing outbreak that had started with the patient
admitted on June 23, not multiple cases. The strain was clearly colonizing
patients “silently”—in ways that avoided detection by throat and groin
cultures. It was also clear that the measures put in place to prevent the
infection’s spread were inadequate. It was time to do more.
When Palmore returned to her office in the Clinical Center
after meeting with Snitkin and Segre, she got another call from the
microbiology lab. A 65-year-old male with lymphoma had just tested
positive for KPC klebsiella. A few weeks earlier, Henderson and Palmore
had recommended that the Clinical Center do something it had never done:
take rectal swabs—which are more likely to detect bacteria than throat or
groin swabs—from every patient in the hospital on an ongoing basis. By the
end of September, four additional colonized patients would be
identified.
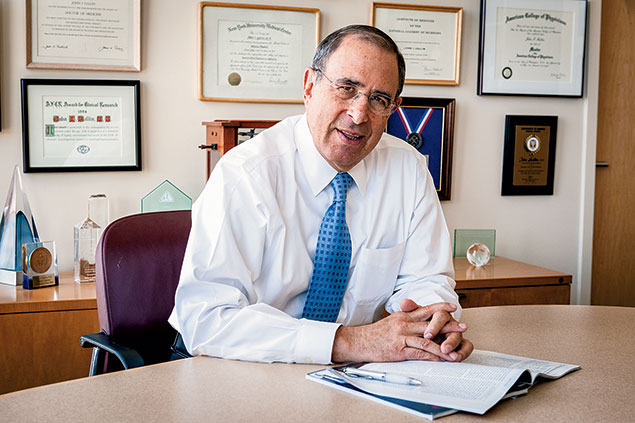
On September 22, a 71-year-old male with lymphoma tested
positive. He was the eighth patient to be colonized; however, he had never
been in the ICU. The bacterium was spreading to other parts of the
hospital.
Staff intensified their efforts to monitor the hospital,
swabbing and culturing handrails and equipment throughout the facility.
They identified KPC klebsiella on a ventilator that had supposedly been
disinfected. At one of the subsequent daily huddles where Palmore
discussed infection-control efforts with hospital staff, someone suggested
a way the Clinical Center could up its game: send in a robot to kill
everything.
The hospital acquired a four-foot-tall robot, called the RBDS,
from Bioquell, a company in Philadelphia. Imagine a version of the Pixar
robot Wall-E without the expressive eyes and with a tank for the torso.
This robot didn’t talk; it fumigated.
The robot was sent into a room, often one filled with medical
equipment. Doorways and ventilation were sealed. Then the robot was
activated. It quickly filled the room with hydrogen-peroxide vapor. The
goal was to saturate every surface and every piece of equipment in the
room, killing everything. Methodically, the Bioquell machine and its
operator moved from room to room through the isolation ICU, eradicating
microorganisms.
But hospitals consist of more than ICUs and patient rooms. In
November, a routine culture found traces of a KPC klebsiella precursor in
a sink drain. Palmore had recently read about transmissions caused by
infected sinks. She contacted the facilities department and requested a
map of the plumbing. Staff removed pipes that Palmore identified as
subjects of concern, disassembling and cleaning each piece with bleach
before reinstalling it.
Nothing seemed to work. The infections kept spreading. On
November 17, a 60-year-old woman with a lung condition tested positive for
KPC klebsiella. She was the 15th patient to be colonized. She had been
admitted to the ICU, spending 12 hours there. The following day another
patient, a 59-year-old man with a mysterious immunodeficiency disorder,
tested positive.
Thanks to DNA sequencing, Palmore and Henderson could determine
exactly who was transmitting the infection to whom (and also rule out the
possibility that a new strain of bacterium had entered the facility). But
they seemed powerless to stop it.
The number of colonized patients threatened to overwhelm the
isolation ward, so the epidemiologists decided to create a new ward by
walling off a passageway—literally. Facilities personnel framed up and
drywalled the old hallway, creating an area for colonized patients with
only one way in or out. Staff identified two other infections in the
following two weeks.
Then a final surprise: The bug disappeared. All told, 18 people
had been infected. Nine patients would die, seven from bloodstream
infections caused by the bacterium, two from underlying illnesses. But by
the end of 2011, the infections stopped. The containment measures finally
appeared to have worked, to the great relief of hospital
staff.
The Clinical Center continued to culture everyone in the
hospital. In early January 2012, staff identified another case of KPC
klebsiella. Snitkin and Segre sequenced it. There was no connection to the
previous year’s outbreak. This bug had come from the outside. In response,
the Clinical Center began testing every new patient admitted to the
facility. In June, staff found another unrelated strain. However, the
transmissions of the original strain had ended. The outbreak appeared to
be over.
• • •
On August 22, 2012, Snitkin, Segre, and several of their
colleagues published a paper in the journal Science Translational
Medicine, entitled “Tracking a Hospital Outbreak of
Carbapenem-Resistant Klebsiella Pneumoniae With Whole-Genome
Sequencing.”
With that article, the Clinical Center revealed to the public
for the first time that it had combatted a dangerous pathogen that had
killed seven people. The response from fellow scientists was enthusiastic.
The reaction from the public was a mixture of fascination and fear.
Parents of children enrolled at Stone Ridge School, across Rockville Pike
from the Clinical Center, called Dr. John Gallin to ask if their children
were safe. (From this particular strain of klebsiella, perfectly, he
assured them.)
But the center was hardly alone in facing such a deadly
bacterium. Surveys conducted by the state of Maryland between 2010 and
2011 found that approximately 80 percent of hospitals in the state had
identified at least one case of carbapenem-resistant enterobacteriaceae
(CRE), such as KPC klebsiella.
To Dr. Lucy Wilson, an epidemiologist for the state of
Maryland, that suggests that CRE “is now endemic in Maryland.” Dr. Alex
Kallen, an infectious-disease expert at the CDC, says KPC klebsiella has
been detected in 42 other states, including Virginia, as well as in the
District.
What was unusual about the Clinical Center’s experience with
KPC klebsiella was not that it had an outbreak but that it quickly
identified it and responded with such vigor. According to epidemiologists,
in many other hospitals the patients would simply have died of an
unspecified bloodstream infection, without anyone ever knowing the precise
cause of their illness or how the infection had spread.
That will likely change. DNA sequencing is rapidly becoming
more affordable. As a result, all hospitals will eventually have access to
the tools that now exist only at NIH and other very specialized hospitals.
However, very few will be able to afford to take the steps NIH did to
contain the outbreak.
There’s another, more sobering, reason people will soon know
more about CREs. Many more patients will almost certainly start to die
from them, because even as we improve our technologies, bacterial defenses
evolve, too.
According to Kallen, the CDC is now receiving reports of
bacteria that are resistant to all existing antibiotics. Doctors
desperately need new, more effective drugs to treat them. But the science
is difficult and the economics of the drug business encourage companies to
focus on treatments for chronic illnesses that require patients to take
medications for years rather than on antibiotics that bacteria will
eventually outwit. “When you look at the timeline for new antibiotics,”
says Kallen, “we are looking at a long time—years.”
Nonetheless, the mood at the National Human Genome Research
Institute, where Segre and Snitkin work, is optimistic. Their research has
provided hospital epidemiologists with an important new tool, and
sequencing holds the potential to give scientists new insights into how
antibiotic resistance evolves.
“In this case,” Segre says, “I would say that when you sequence
every single base pair of its 5 million base pairs, the bacteria has no
secrets left.”
Scientists hope these insights will translate into more
effective drugs. But while researchers at NIH may have stripped KPC
klebsiella of its secrets, that doesn’t mean they’re on the verge of
mastering the microcosm. They haven’t even mastered this
strain.
Last April, the Clinical Center admitted a young man from
Minnesota to receive treatment for complications arising from a
bone-marrow transplant. The patient was suffering from a severe case of
graft-versus-host disease, in which the transplanted cells attack the
body.
In this dangerously immunocompromised state, he came down with
a case of pneumonia caused by a different bacterium, Pseudomonas
aeruginosa, known for its ability to survive outside the body on
surfaces in hospitals and in medical equipment. The patient
survived.
Then he tested positive for KPC klebsiella. Snitkin and Segre
immediately sequenced the genome. It was the same strain that had first
arrived in the hospital a year earlier, in June 2011. Despite NIH’s
exhaustive efforts to exterminate it, the original strain of KPC lived
on.
On September 7, 2012, the young man died in the isolation
ward.
Several days later, NIH staff swabbed and cultured a handrail
outside his room. They found the bug.
John Buntin (jbbuntin@gmail.com) is a staff correspondent at
Governing magazine and author of “LA Noir: The Struggle for the Soul of
America’s Most Seductive City.”
This article appears in the June 2013 issue of The Washingtonian.